Active matter describes out-of-equilibrium systems that consist of components that locally convert energy into motion, such as schooling fish and flocking birds, as well as, on smaller scales, swimming bacteria and crawling cells. Nature has perfected obtaining robust collective behavior and global order from simple local interactions. A great challenge is to engineer similar systems at all scales and in different environments, that are composed of many agents, ranging from self-propelled nanoparticles in solution to self-driving cars in traffic, and to be able to control their emergent collective properties, their emergent “intelligence”.
We believe computer simulations have the unique opportunity and responsibility to lead the way in searching for, understanding, controlling and designing these new materials that are catalysts for technological innovation.
We are always excited to talk to prospective collaborators, postdocs, and students! Explore our research further by reading about our research topics below.
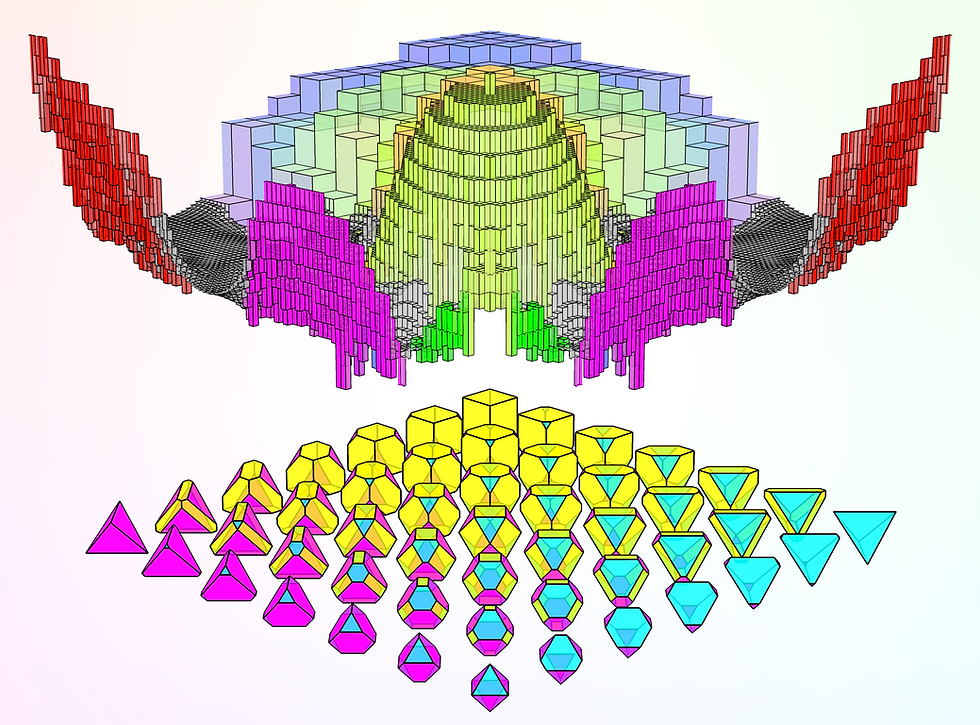
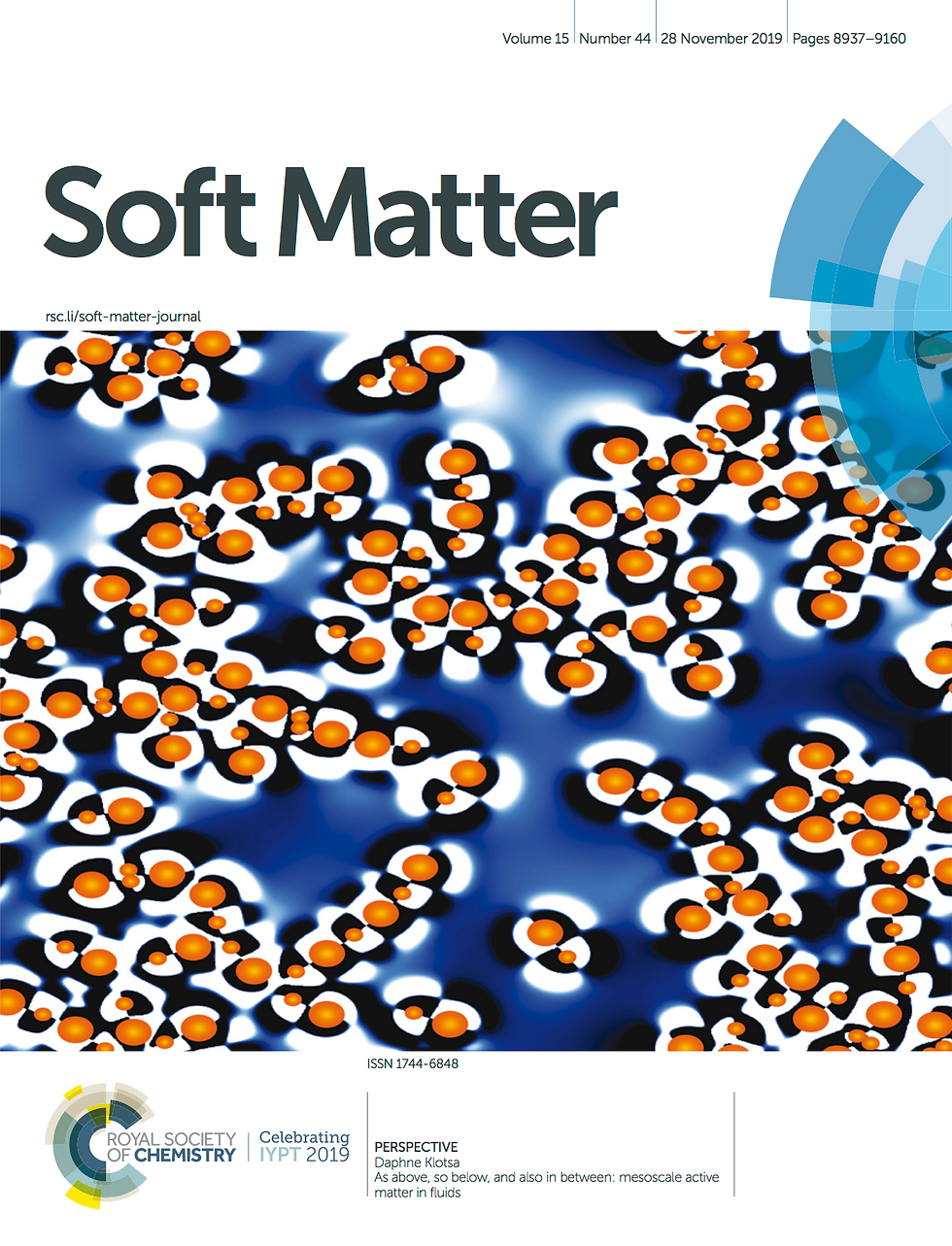
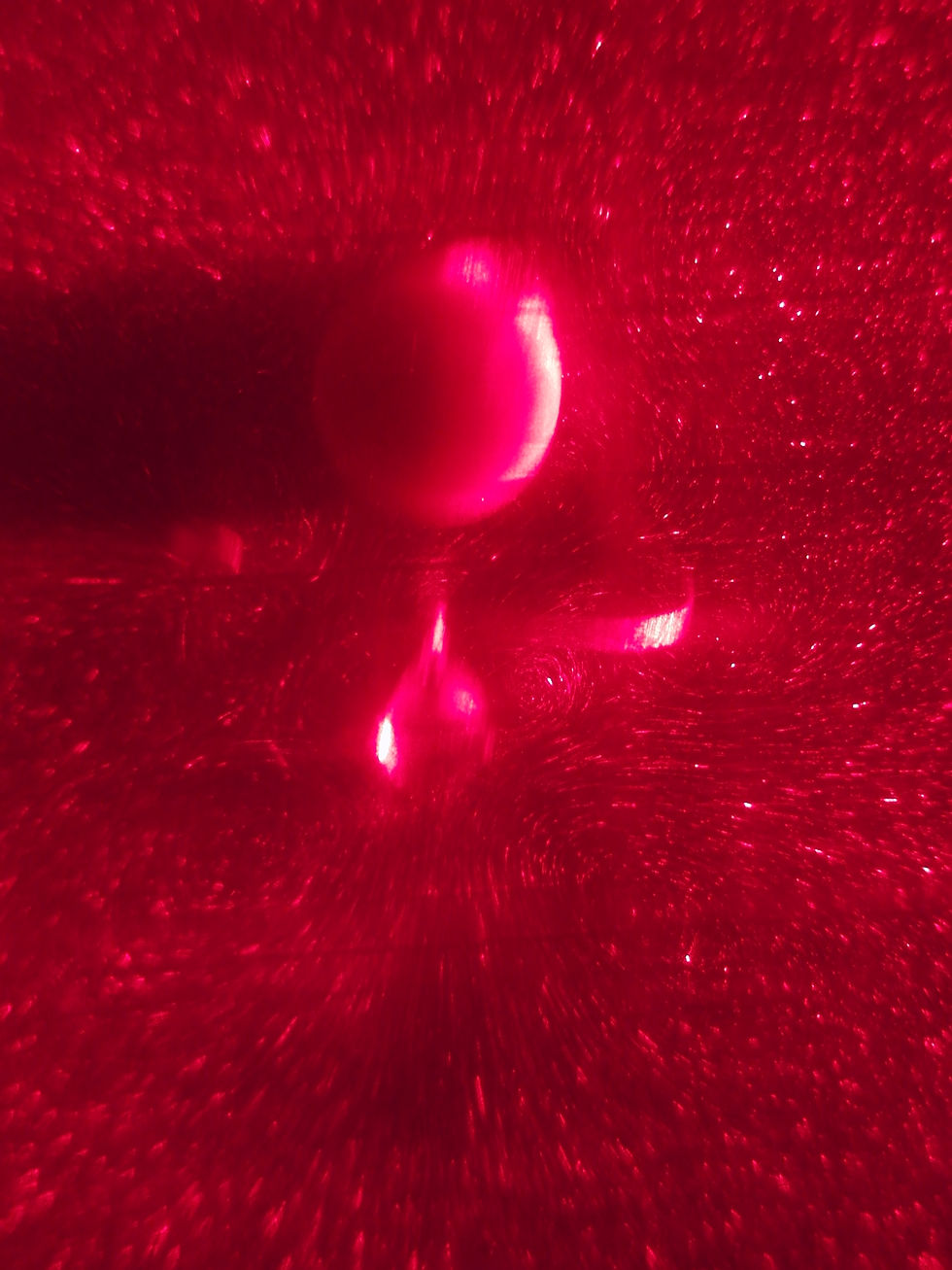
around little two-sphere swimmer. From PRL (2016).
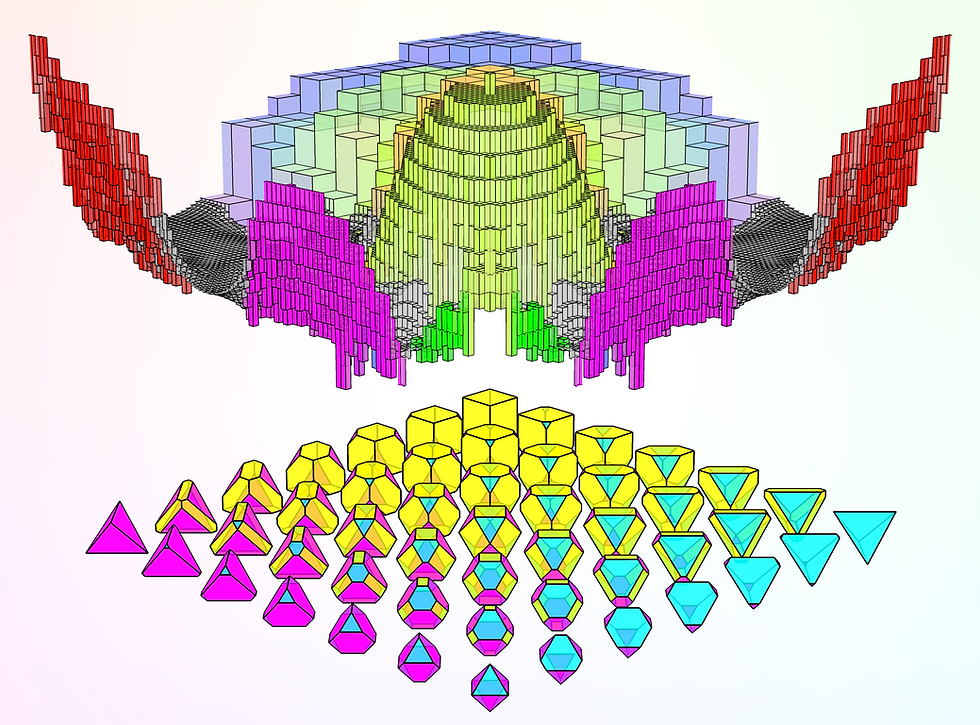
Research Topics
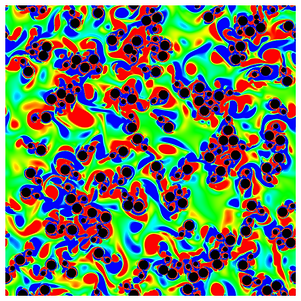
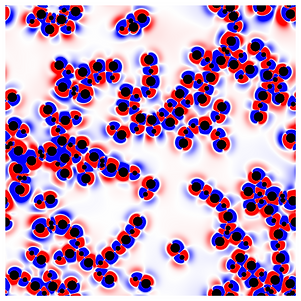
Mesoscale active matter in fluids
Living matter, such as biological tissue, can be viewed as a nonequilibrium hierarchical assembly, where at each scale self-driven components come together by consuming energy in order to form increasingly complex structures. The remarkable properties of living or ‘‘active-matter’’ systems, as they are generally known, such as versatility, self-healing, and self-replicating, have prompted the following questions: (1) do we understand the biology and biophysics that give rise to these properties? (2) can we achieve similar functionality with synthetic active materials? We are particularly interested in active matter systems in fluids with finite inertia. Finite inertia is relevant for mesoscale organisms that swim or fly covering at least three orders of magnitude in size (~0.5 mm–50 cm) and their collective behavior is generally unknown. As a result, we are limited both in our understanding of the biology of mesoscale swarms and processes but also in our design of self-powered machines and robots at those scales. We expect interesting collective behavior to emerge because with finite inertia, come nonlinearities and the many-body hydrodynamic interactions between the organisms/particles can become quite complex, potentially leading to phenomena, such as novel flocking states and nonequilibrium phase transitions that have not been observed before and which could have great impact in materials applications.
-
Publications:
-
As Above, So Below, and also in Between: Mesoscale active matter in fluids. D. Klotsa. Soft Matter (invited perspective) 15, 8946 (2019).
-
Transition in motility mechanism due to inertia in a model self-propelled two-sphere swimmer. T. Dombrowski et al., Phys. Rev. Fluids (Rapid Comms) 4, 021101(R) (2019).
-
Propulsion of a Two-Sphere Swimmer. D. Klotsa et al., PRL 115, 248102 (2015).
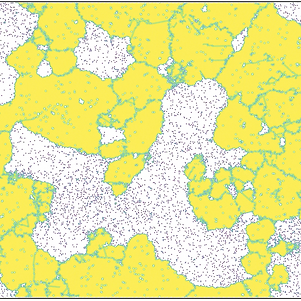
Multicomponent active matter
We investigate collective phenomena with active particles, and particularly heterogeneous systems where there are more than one species of active particles. For example, we studied rotationally driven spinners of concave shape, where each spinner experiences a constant internal torque in either a clockwise or counterclockwise direction. Although the spinners are modeled as hard, otherwise non-interacting rigid bodies, we found that their active motion induces an effective interaction that favors rotation in the same direction. They thus phase separate/demix as if they were oil and water. We are now investigating systems with mixtures of self-propelled particles, imagine for example two species of fish, if they were close to one another, would they form two separate schools, would they mix, would they form one big school and under what conditions?
-
Publications:
-
Phase behavior and surface tension of soft active Brownian particles. N. Lauersdorf, T. Kolb, M. Moradi, E. Nazockdast and D. Klotsa. 2021 Soft Matter Emerging Investigators themed collection (invited) Soft Matter 17, 6337-6351 (2021).
-
Active binary mixtures of fast and slow hard spheres. T. Kolb and D. Klotsa. Soft Matter 16, 1967 (2020).
-
Shape control and compartmentalization in active colloidal cells. M. Spellings et al. PNAS 112, no. 34, E4642–E4650 (2015).
-
Emergent collective phenomena in a mixture of hard shapes through active rotation. N.H.P. Nguyen et al. PRL 112, 075701 (2014).
-
Press: Active colloids: Made to order. A. Klopper, Nature Physics 11, 703 (2015).
-
University of Michigan news.

Pattern formation of spheres in oscillating fluids
Rigid spherical particles in oscillating fluid flows form interesting patterns as a result of fluid mediated interactions. Here, through both experiments and simulations, we show that two spheres under horizontal vibration align themselves at right angles to the oscillation and sit with a gap between them, which scales in a non-classical way with the boundary layer thickness. A large number of spherical particles form strings perpendicular to the direction of oscillation. Investigating the details of the interactions we find that the driving force is the nonlinear hydrodynamic effect of steady streaming. We then design a simple swimmer (two-spheres-and-a-spring) that utilizes steady streaming in order to propel itself and discuss the nature of the transition at the onset of swimming as the Reynolds number gradually increases.
-
Publications:
-
Chain formation of spheres in oscillatory fluid flows. D. Klotsa et al. PRE 79, 021302 (2009).
-
The interaction of spheres in oscillatory fluid flows. D. Klotsa et al. PRE 76, 056314 (2007).
-
Video:
-
CSAWW at the University of Michigan.
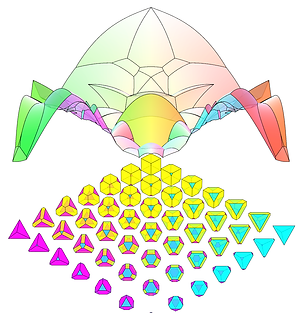
Packing and self-assembly
What is the best way to pack objects in space? Dense packings of hard polyhedra have been studied for centuries due to their mathematical aesthetic and more recently for their applications in fields such as granular matter, amorphous matter, and biology. The spontaneous organization of hard polyhedra in solution has only recently been addressed, demonstrating a plethora of assembled complex structures. For any given shape, the densest packings and the structures assembled spontaneously from the fluid are often different. We investigate connections between packings and assemblies for families of convex polyhedra. We investigate the possibility of predicting one from knowledge of the other, discuss trends, and link with previous works.
-
Publications:
-
Intermediate crystalline structures of colloids in shape space. Klotsa et al., Soft Matter 2018, DOI: 10.1039/C8SM01573B, (2018).
-
Clusters of polyhedra in spherical confinement. E. G. Teich, G. van Anders, D. Klotsa et al. PNAS 113, no 6, E669-678 (2016).
-
Complexity in surfaces of densest packings for families of polyhedra. E. R. Chen*, D. Klotsa*, et al. Phys. Rev. X 4, 011024 (2014). *contributed equally.
-
Understanding shape entropy through local dense packing. G. van Anders, D. Klotsa, et al. PNAS 111, no 45, E4812--E4821 (2014).
-
Press: physics.org (clusters), Synopsis, physicsworld and the New Scientist, "Material witness: The force of shape". P. Ball, Nature Materials 13, 1083 (2014) and physics.org (entropy).
-
Video: Public talk at Columbia.